Short Introduction for Microneedles
Over the past few decades, significant research has focused on the development and application of microneedle arrays (MNAs) for drug delivery. MNAs are minimally invasive systems that offer several advantages over conventional drug delivery methods, such as hypodermic injections and oral medications. The skin serves as an optimal target due to its high concentration of antigen-presenting cells, which can effectively stimulate immune responses. This enables the absorption of bioactive molecules from the skin’s vascular-rich microenvironment [1,2]. Furthermore, MNAs provide benefits such as targeted delivery, improved storage conditions, ease of use, and reduced incidents of application errors and side effects [3,4].
Microneedle arrays are classified into various categories based on their morphology, including hollow, solid, coated, dissolving, and swellable MNAs [5]. Selecting the appropriate type of microneedle (MN) depends on the intended application [6-8]. According to the 2014 Roots Analysis report, it is estimated that between 2014 and 2030, over 70% of MNAs developed will feature solid or dissolving/soluble needles, reflecting their versatility in drug delivery [9]. Among these, dissolving MNs are particularly advantageous due to the wide range of biocompatible materials available for their fabrication, allowing for efficient drug delivery. As a result, dissolving microneedles constitute the primary focus of research on using MNs for delivering therapeutics in arthritis treatment [10].
The production and application of MNAs require careful consideration of material properties based on their type. For instance, fast-dissolving MNs for arthritis management have been developed using materials such as polyvinyl alcohol (PVA) and poly(vinyl pyrrolidone) (PVP) [11]. PVP/HA-loaded MNs demonstrated improved bioavailability and performance comparable to or better than oral treatments for osteoarthritis [12]. MNs made from a combination of chitosan (CS), PVP, and carboxymethyl cellulose (CMC) showed excellent biocompatibility, deep skin penetration, and significant reduction in joint swelling, offering promising therapeutic outcomes for arthritis [13]. Similarly, MNs composed of poly(lactide-co-glycolide) (PLGA) effectively reduced synovial inflammation and angiogenesis, exhibiting strong anti-inflammatory effects in arthritic rats [14]. Once these needles penetrate the outer skin layer, they dissolve, delivering biological materials directly to the subcutaneous microenvironment [15]. Recently, research on dissolving MNAs tip-loaded with chondroitin sulfate (CS), glycosaminoglycan (GAG), and hyaluronic acid (HA) showed significant improvement of the cell proliferation for hFOB and HC cell lines [16].
To fabricate the MNAs, generally a two-step process is employed. The first step is the fabrication of master molds, and the second step is the fabrication of MNAs using the mastermolds. Figure 1 illustrates an example of this two-step fabrication.
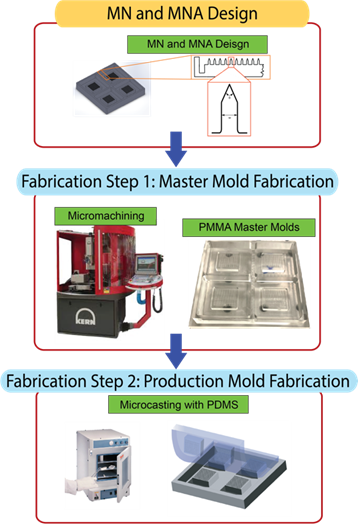
Figure 1. Master & Production Mold Fabrication [16].
In the first step, master (male) molds made of poly(methyl methacrylate) (PMMA) were created using mechanical micro-manufacturing techniques [17]. To ensure cleanliness and remove any residual debris, the molds were subjected to ultrasonic cleaning in a bath set to 50 °C for 45 minutes. In the second step, production (female) molds were prepared using polydimethylsiloxane (PDMS). The PDMS base and curing agent were mixed in a 10:1 weight ratio. During mixing, air became trapped in the mixture, forming micro-bubbles. To eliminate these bubbles, the mixture underwent degassing in a desiccator, with repeated cycles of vacuum application and release. The degassed mixture was then poured into the master mold and cured in an oven at 75 °C for one hour. After cooling the cured molds to room temperature, they were carefully separated from the master mold. An example of fabricated MNAs is shown below where the images show that the MNs, in arrays or individually, retain their exact geometry and morphology without structural distortion.
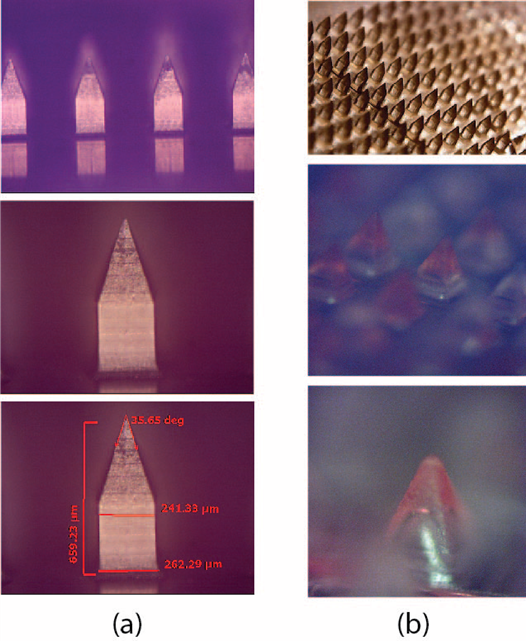
Figure 2. a) Light microscopy images (LIMI) of the fabricated MNs: the top row shows a wide-angle view, the middle row provides a focused image of a single needle, and the bottom row illustrates an example of the characterization measurements conducted. (b) MNA and bio-cargo-loaded MN tips: the top row presents a wide-angle optical microscope image, the middle row offers a closer-field view, and the bottom row focuses on the tip of a single needle [16].
References
[1] Dahmer S, Schiller RM. Glucosamine. American Family Physician. 2008;78(4):471–476.
[2] Uebelhart D. Clinical review of chondroitin sulfate in osteoarthritis. Osteoarthritis Cartilage. 2008;16 Suppl 3(Suppl. 3):S19–S21. doi: 10.1016/j.joca.2008.06.006.
[3] Miller LE, Fredericson M, Altman RD. Hyaluronic acid injections or oral nonsteroidal anti-inflammatory drugs for knee osteoarthritis: systematic review and meta-analysis of randomized trials. Orthop J Sports Med. 2020;8(1):2325967119897909. doi: 10.1177/2325967119897909.
[4] Prausnitz MR, Langer R. Transdermal drug delivery. Nat Biotechnol. 2008;26(11):1261– 1268. doi: 10.1038/nbt.1504.
[5] Sharma A, Arora S. Dermal delivery of glucosamine sulphate: formulation, characterization and performance evaluation. World J Pharm Pharm Sci. 2013;2(6):6448–6462.
[6] Moffatt K, Wang Y, Raj Singh TR, et al. Microneedles for enhanced transdermal and intraocular drug delivery. Curr Opin Pharmacol. 2017;36:14–21. doi: 10.1016/j. coph.2017.07.007.
[7] Liu GS, Kong Y, Wang Y, et al. Microneedles for transdermal diagnostics: recent advances and new horizons. Biomaterials. 2020;232:119740. doi: 10.1016/j.biomaterials.2019.119740.
[8] Duarah S, Sharma M, Wen J. Recent advances in microneedle-based drug delivery: special emphasis on its use in paediatric population. Eur J Pharm Biopharm. 2019;136:48–69. doi: 10.1016/j.ejpb.2019.01.005.
[9] Tissone L. The rapid evolution of the transdermal drug delivery industry spawns innovation. 2016;65:14–16.
[10] Wang J, Zeng J, Liu Z, et al. Promising strategies for transdermal delivery of arthritis drugs: microneedle systems. Pharmaceutics. 2022;14(8):1736. doi: 10.3390/pharmaceutics14081736.
[11] Amodwala S, Kumar P, Thakkar HP. Statistically optimized fast dissolving microneedle transdermal patch of meloxicam: a patient friendly approach to manage arthritis. Eur J Pharm Sci. 2017;104:114–123. doi: 10.1016/j.ejps.2017.04.001.
[12] Wang Q, Yang X, Gu X, et al. Celecoxib nanocrystal-loaded dissolving microneedles with highly efficient for osteoarthritis treatment. Int J Pharm. 2022;625:122108. doi: 10.1016/j.ijpharm.2022.122108.
[13] Yao W, Tao C, Zou J, et al. Flexible two-layer dissolving and safing microneedle transdermal of neurotoxin: a biocomfortable attempt to treat rheumatoid arthritis. Int J Pharm. 2019;563:91–100. doi: 10.1016/j.ijpharm.2019.03.033.
[14] Hu H, Ruan H, Ruan S, et al. Acid-responsive pegylated branching PLGA nanoparticles integrated into dissolving microneedles enhance local treatment of arthritis. Chemical Engineering Journal. 2022;431:134196. doi: 10.1016/j.cej.2021.134196.
[15] Arora A, Prausnitz M, Mitragotri S. Micro-scale devices for transdermal drug delivery. Int J Pharm. 2008;364(2):227–236. doi: 10.1016/j.ijpharm.2008.08.032.
[16] Choupani, A., Temucin, E. S., Ciftci, E., Bakan, F., Camic, B. T., Ozkoc, G., … Bediz, B. (2024). Design of poly(vinyl pyrrolidone) and poly(ethylene glycol) microneedle arrays for delivering glycosaminoglycan, chondroitin sulfate, and hyaluronic acid. Journal of Biomaterials Science, Polymer Edition, 36(1), 64–85. https://doi.org/10.1080/09205063.2024.2392914.
[17] Bediz B, Korkmaz E, Khilwani R, et al. Dissolvable microneedle arrays for intradermal delivery of biologics: fabrication and application. Pharm Res. 2014;31(1):117–135. doi: 10.1007/s11095-013-1137-x.